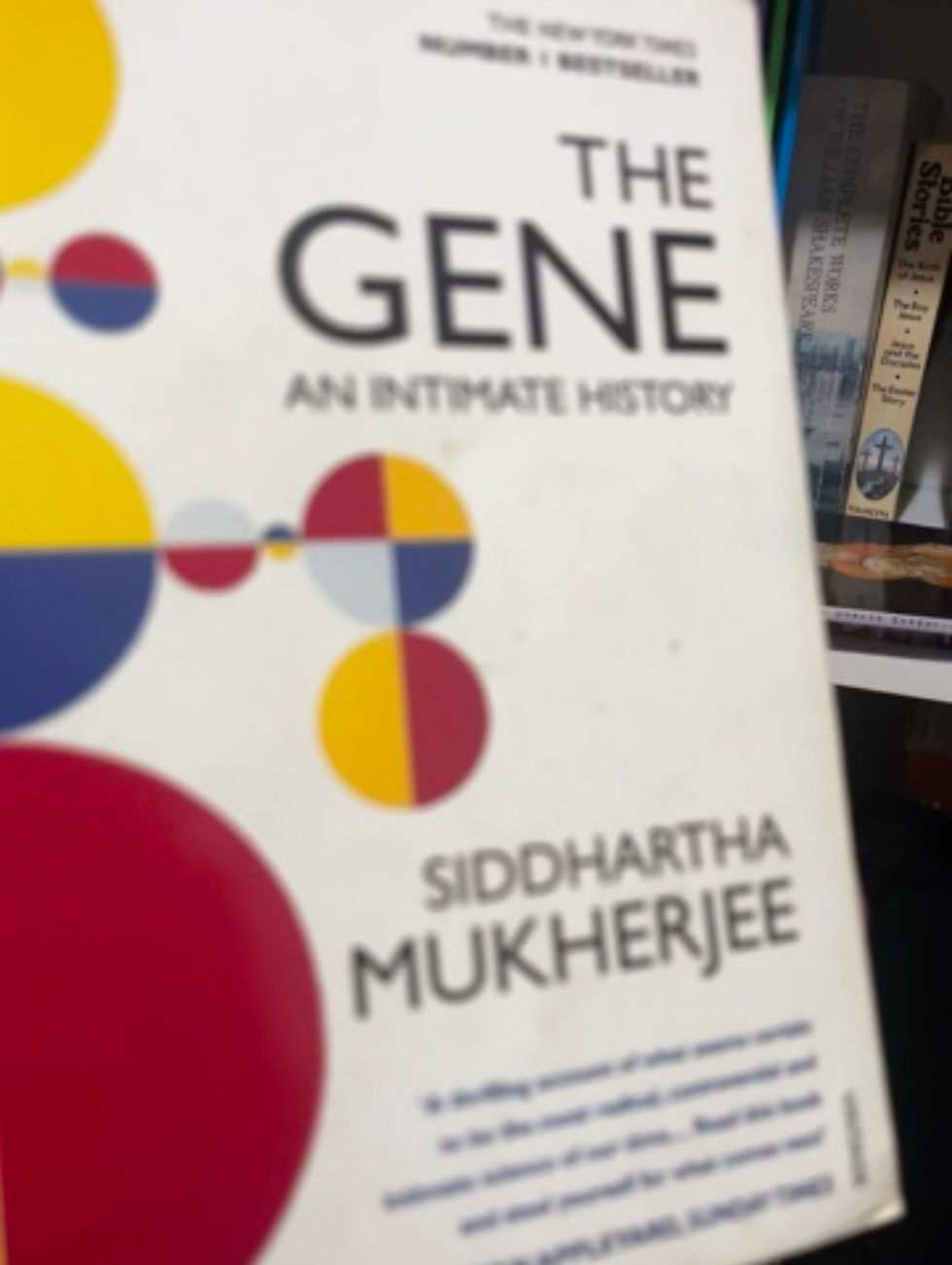
Abstract
The concept of discrete hereditary units, first conceptualised as “factors” by Gregor Mendel and later formalised as genes, represents one of the most transformative ideas in biology. This article explores the history and molecular basis of these units, emphasising their role in botany. From Hugo de Vries’ mutation theory to modern plant genomics, the understanding of gene structure, regulation, and expression has profoundly influenced agriculture, ecological conservation, and responses to environmental challenges. Drawing on peer-reviewed research, this paper provides an advanced perspective on the foundational discoveries and their implications for plant science and dependent systems such as ecosystems and human populations.
1. Introduction
The inheritance of traits has fascinated scientists for centuries, but it was not until the mid-19th century that Gregor Mendel demonstrated that inheritance occurs through discrete units, later named genes. This principle, rediscovered and expanded upon by Hugo de Vries, has since evolved into a cornerstone of modern biology. In botany, understanding the mechanisms of gene function and expression has enabled breakthroughs in plant breeding, genetic modification, and conservation. This article investigates the molecular foundations of hereditary units and their applications, highlighting their impact on plants and their dependents.
2. Historical Context
2.1 Gregor Mendel: The Pioneer of Genetics
In 1866, Gregor Mendel published the results of his experiments on Pisum sativum (pea plants), where he identified that traits such as seed shape and flower colour are inherited in predictable patterns.
He described the “laws of inheritance”:
• Law of Segregation: Alleles separate during gamete formation.
• Law of Independent Assortment: Traits are inherited independently if on separate chromosomes.
• Law of Dominance: Certain traits mask others in heterozygous pairings.
Although Mendel’s work was initially overlooked, his findings formed the basis of modern genetics.
2.2 Rediscovery and Hugo de Vries’ Mutation Theory
In 1900, Hugo de Vries, along with Carl Correns and Erich von Tschermak, independently rediscovered Mendel’s principles. De Vries expanded upon these findings by proposing the mutation theory, which suggested that sudden, large-scale changes in genetic material could give rise to new species. His work on Oenothera lamarckiana (evening primrose) provided critical insights into genetic variation, though his conclusions were later refined with the integration of Mendelian genetics into the modern synthesis of evolution.
3. Molecular Basis of Discrete Hereditary Units
3.1 DNA as the Foundation of Heredity
The landmark discovery by Avery, MacLeod, and McCarty in 1944, identifying DNA as the hereditary material, and Watson and Crick’s 1953 elucidation of its double-helix structure, established the molecular basis of genes. A gene is now defined as a segment of DNA encoding functional molecules, such as proteins or regulatory RNAs. These discoveries reinforced the concept of discrete hereditary units first posited by Mendel and later expanded by de Vries.
3.2 Gene Expression Mechanisms
Gene expression involves multiple regulatory layers that determine when and how genetic information is used:
1. Transcriptional Regulation: Transcription factors interact with specific DNA sequences to modulate gene activity. Advances in plant genomics have revealed regulatory networks, with tools like ChIP-seq elucidating the binding sites of transcription factors (Zhu et al., 2019).
2. Epigenetic Regulation: Modifications such as DNA methylation and histone acetylation influence gene expression without altering the DNA sequence. Research into plants has identified over 130 genes involved in epigenetic control (Liu et al., 2021), which play a role in stress responses and development.
3. Post-Transcriptional Regulation: Mechanisms such as RNA splicing and silencing contribute to gene diversity and regulation. Small RNAs like miRNAs are crucial for regulating developmental genes and stress responses in plants (Zhang et al., 2020).
4. Post-Translational Regulation: Protein modifications, including phosphorylation, impact the stability and function of gene products, fine-tuning the cellular response.
4. Impact in Botany
4.1 Advances in Plant Breeding and Agriculture
The application of Mendelian genetics revolutionised agriculture:
• The Green Revolution leveraged hybridization and mutation breeding to develop high-yield crops such as semi-dwarf wheat (Borlaug, 1968).
• Genetic engineering introduced traits like drought resistance (e.g., DREB1A in rice) and pest resistance (Bt cotton), significantly improving productivity (James, 2021).
4.2 Conservation and Climate Resilience
Understanding genetic diversity helps conserve endangered species and adapt plants to climate change. For instance, introducing C4 photosynthesis traits into rice aims to increase efficiency under heat stress (von Caemmerer et al., 2012).
5. Broader Implications
5.1 Ecosystems
Genetic diversity in plants stabilises ecosystems. Monocultures, such as those that led to the Irish Potato Famine, highlight the dangers of reduced genetic variability.
5.2 Human Societies
Crops like golden rice address nutritional deficiencies, demonstrating the societal importance of genetic research in plants (Beyer et al., 2002).
6. Challenges and Future Directions
Despite advancements, ethical concerns about genetic modification persist. Responsible regulation and public education are essential to harness the benefits of plant genetics sustainably.
7. Conclusion
The concept of discrete hereditary units has transformed our understanding of biology and revolutionised botany. From Mendel’s experiments to Hugo de Vries’ mutation theory and modern molecular biology, these insights have driven progress in agriculture, conservation, and ecological resilience.
References
1. Avery, O. T., MacLeod, C. M., & McCarty, M. (1944). Studies on the chemical nature of the substance inducing transformation of pneumococcal types. Journal of Experimental Medicine, 79(2), 137-158.
2. Watson, J. D., & Crick, F. H. C. (1953). Molecular structure of nucleic acids: A structure for deoxyribose nucleic acid. Nature, 171(4356), 737-738.
3. Liu, S., et al. (2021). Epigenetic mechanisms in plant stress responses. Annual Review of Plant Biology, 72, 567-593.
4. Borlaug, N. E. (1968). Wheat breeding and its impact on world food supply. Science, 162(3853), 967-969.
5. von Caemmerer, S., & Furbank, R. T. (2012). Strategies for improving C4 photosynthesis. Journal of Experimental Botany, 63(1), 67-75.
6. Beyer, P., et al. (2002). Golden rice: Introducing the beta-carotene biosynthetic pathway into rice endosperm. Science, 287(5451), 303-305.
7. James, C. (2021). Global status of commercialized biotech crops. ISAAA Briefs.
Comments