Reaction Kinetics: Bridging Molecular Mechanisms and Real-World Innovations The Dynamic Interplay Between Chemical Rates, Life Processes, and Human-Inspired Solutions
- amuggs82
- Dec 22, 2024
- 5 min read
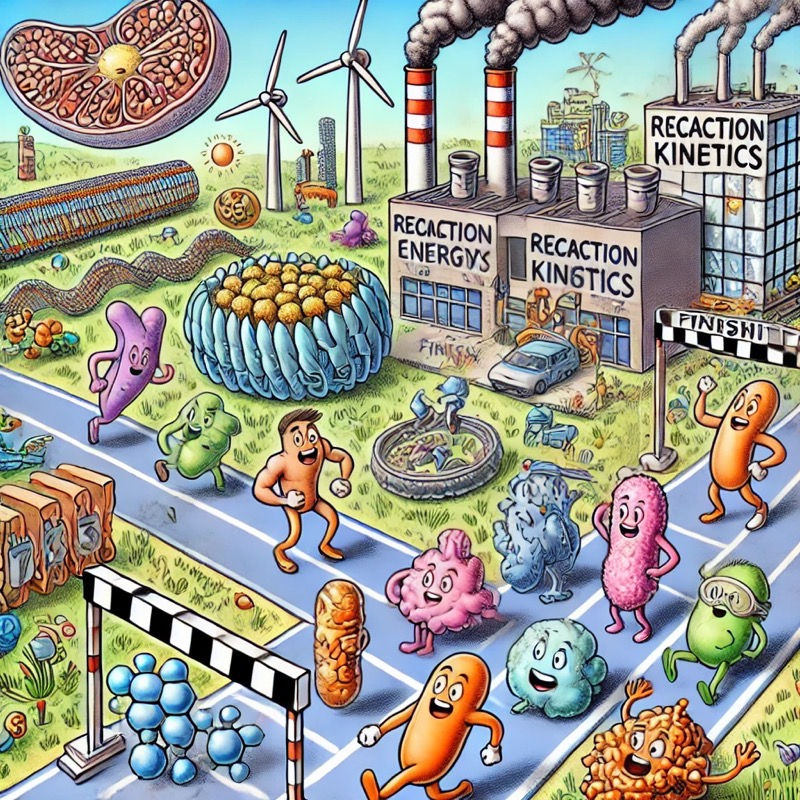
Abstract
Reaction kinetics, the study of the speed and mechanisms of chemical reactions, is a critical field with far-reaching implications. From fueling life through cellular respiration to shaping real-world applications in sustainable technologies, reaction kinetics provides a framework for understanding and manipulating energy and matter. This article explores reaction kinetics in the context of cellular respiration while connecting its principles to real-world applications such as industrial catalysis, renewable energy systems, and biomimetic architecture. By integrating theoretical insights with applied science, the article seeks to educate and inspire curiosity about this essential yet underappreciated discipline.
Introduction: Why Reaction Kinetics Matters
Imagine a race where molecules are the runners, and the goal is transformation—this is reaction kinetics. However, unlike a simple footrace, the factors that govern molecular speed, direction, and success are complex, including energy barriers, catalysts, and environmental influences. Reaction kinetics provides the theoretical and experimental tools to analyse these processes, offering insights not only into fundamental scientific principles but also into technologies that impact daily life.
This article explores reaction kinetics in two interconnected dimensions:
1. Its role in life-sustaining biochemical processes like cellular respiration.
2. Its applications in addressing pressing challenges, such as climate change, energy efficiency, and sustainable design.
Through these lenses, we illustrate how reaction kinetics is both a cornerstone of science and a driver of innovation.
Reaction Kinetics in Action: Cellular Respiration
Cellular respiration, a cornerstone of biochemistry, demonstrates how nature manages reaction kinetics with precision. This process, essential to life, converts glucose into ATP, enabling cells to perform functions ranging from muscle contraction to neural signaling.
1. Glycolysis: The Gateway to Energy
The first stage of cellular respiration, glycolysis, breaks down glucose into pyruvate in a series of enzyme-catalysed steps. Each reaction has a specific rate determined by substrate concentration, enzyme availability, and feedback inhibition. For instance, phosphofructokinase (PFK), a key enzyme in glycolysis, is regulated by ATP levels, ensuring the pathway operates efficiently under varying energy demands.
2. The Krebs Cycle: A Metabolic Hub
In the mitochondrial matrix, the Krebs cycle (or citric acid cycle) transforms acetyl-CoA into carbon dioxide while transferring electrons to carrier molecules like NADH and FADH₂. The kinetic efficiency of these reactions ensures that cells maximise energy extraction from nutrients, a principle with direct implications for industrial bioprocessing.
3. Oxidative Phosphorylation: Kinetics Meets Thermodynamics
The final stage of cellular respiration involves the electron transport chain, where electrons flow through protein complexes, driving proton pumping to create an electrochemical gradient. ATP synthase harnesses this gradient to produce ATP, the universal energy currency. Here, the tight coupling of reaction rates to thermodynamic efficiency offers lessons for energy storage and conversion technologies.
From Cells to Systems: Applied Reaction Kinetics
Cellular respiration is a remarkable biological process, but reaction kinetics also fuels innovation in applied sciences. By adapting concepts like energy efficiency, catalysis, and flexibility from biology to technology, researchers are addressing practical challenges and finding solutions.
1. Industrial Catalysis: Learning from Enzymes
Catalysts are central to reaction kinetics, lowering activation energy to speed up chemical processes. In industrial settings, catalysts are used to manufacture essential chemicals, reduce waste, and minimise energy consumption.
• Haber-Bosch Process: This reaction for ammonia synthesis from nitrogen and hydrogen is kinetically limited under normal conditions. Catalysts, such as iron with potassium promoters, accelerate the reaction, enabling large-scale fertiliser production.
• Green Catalysis: Inspired by enzymes, researchers are developing biomimetic catalysts that operate under mild conditions, reducing environmental impact. Examples include photocatalysts for CO₂ reduction, mimicking the natural carbon fixation in plants.
2. Renewable Energy Systems
Reaction kinetics underpins technologies that convert and store energy efficiently:
• Fuel Cells: The kinetics of hydrogen oxidation and oxygen reduction dictate the efficiency of fuel cells, which produce electricity cleanly. Advances in catalyst design aim to improve these rates, bringing fuel cells closer to mainstream adoption.
• Batteries: In lithium-ion batteries, the rate of lithium ion intercalation into electrodes is a kinetic bottleneck. Optimising these reaction rates improves charge times and battery lifespans, directly impacting renewable energy storage.
3. Biomimetic Architecture: Building with Kinetic Wisdom
The principles of reaction kinetics also inspire sustainable design. Biomimetic architecture, which emulates nature’s strategies, demonstrates how kinetic principles enhance energy efficiency and adaptability:
• Termite Mounds and Passive Cooling: Termite mounds maintain stable internal temperatures by optimising the kinetics of air and heat flow. Buildings like the Eastgate Centre in Zimbabwe mimic this mechanism, drastically reducing energy use for cooling.
• Self-Healing Materials: Inspired by biological repair processes, materials such as self-healing concrete incorporate chemical reactions that activate upon damage, extending infrastructure lifespan.
Observable Real-World Impacts
The relevance of reaction kinetics extends beyond laboratories and design studios into the observable, everyday world:
• Healthcare: Understanding reaction kinetics is critical in pharmacology. The rate at which drugs are metabolised (pharmacokinetics) determines their efficacy and safety. For instance, slow-acting drugs can lead to accumulation and toxicity, while fast-acting ones may require frequent dosing.
• Environmental Chemistry: The kinetics of pollutant breakdown, such as photodegradation of plastics or the decomposition of greenhouse gases, directly influences environmental sustainability. Advances in this field are paving the way for cleaner air and water.
• Food Science: Reaction kinetics plays a role in processes like fermentation, cooking, and spoilage. The Maillard reaction, responsible for browning and flavor development, is a kinetic process that chefs and food technologists manipulate for culinary excellence.
The Future of Reaction Kinetics
The study of reaction kinetics is entering a transformative era. Computational modeling, machine learning, and high-throughput experimentation are expanding our ability to predict and control reaction rates. Moreover, interdisciplinary research is unlocking new applications:
• Artificial Photosynthesis: Kinetic optimisation is key to mimicking natural photosynthesis for solar fuel production.
• Synthetic Biology: Engineering metabolic pathways requires precise control of reaction kinetics to balance fluxes and avoid bottlenecks.
• Climate Mitigation: Kinetic models of carbon capture reactions are enabling the design of more efficient sequestration technologies.
Conclusion: Reaction Kinetics as a Driver of Curiosity and Change
Reaction kinetics is far more than an abstract scientific concept—it is a dynamic force shaping the natural and engineered worlds. By understanding how molecules interact and transform, we gain the tools to tackle challenges ranging from energy efficiency to environmental sustainability. Moreover, drawing inspiration from natural systems like cellular respiration enriches our ability to design innovative technologies that harmonise with the world around us.
The study of reaction kinetics invites us to see the invisible: the molecular motions that govern life, power technology, and inspire creativity. It is a field ripe for exploration, offering profound insights and practical applications that can transform industries and improve lives.
References
1. Alberts, B., Johnson, A., Lewis, J., et al. (2002). Molecular Biology of the Cell. New York: Garland Science.
2. Ertl, G. (2008). “Reactions at Solid Surfaces.” Nobel Lecture in Chemistry.
3. Turner, J. S. (2000). The Extended Organism: The Physiology of Animal-Built Structures. Cambridge, MA: Harvard University Press.
4. Biomimicry Institute. (2020). “Nature-Inspired Innovation.” Retrieved from biomimicry.org.
5. Hammes-Schiffer, S. (2015). “Proton-Coupled Electron Transfer: Moving Together and Charging Forward.” Accounts of Chemical Research, 48(9), 2719–2726.
This article aspires to make reaction kinetics accessible and engaging, fostering curiosity among students, scientists, and innovators alike. Reaction kinetics is not just about the speed of change; it is about understanding the nature of transformation.
Comments