[Biomimetic Design] Fictional Physics and Scientific Reality: From Flux Capacitors to Silicon Dies
- amuggs82
- Feb 1
- 4 min read
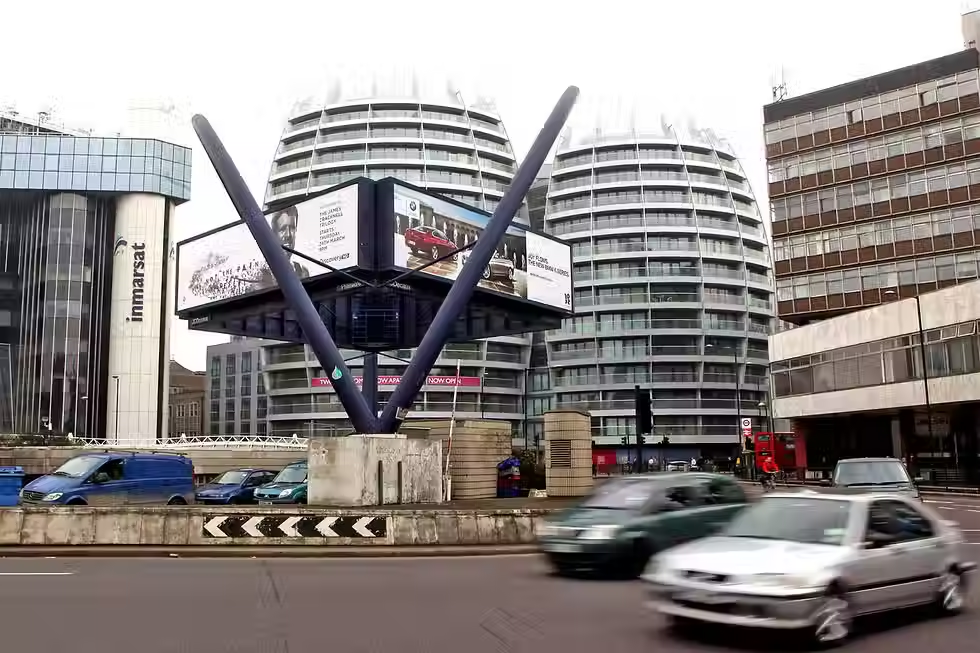
City in the cloud: London’s Tech City, pictured, could be pricing out small firms and helping the growth of clusters in other parts of the country (Getty Images)
Abstract
Fictional devices such as the flux capacitor in Back to the Future offer a gateway to explore the boundaries between imaginative concepts and scientific plausibility. This article examines the flux capacitor through the lens of established physics principles, connects it to phenomena such as Brownian motion and semiconductor physics, and illustrates how interdisciplinary science bridges fictional concepts to real-world technologies. By integrating thermodynamics, quantum mechanics, and material science, we reveal a continuum of energy manipulation and control that ties together seemingly disparate phenomena.
Introduction
Science fiction often stretches the imagination by presenting technologies rooted in speculative or fictional physics. Among the most iconic examples is the flux capacitor, introduced in the 1985 film Back to the Future, described as enabling time travel when powered by 1.21 gigawatts. While this device remains firmly in the realm of fiction, its conceptual underpinnings invite discussions that blur the line between imagination and scientific theory.
Through a detailed exploration of relevant scientific principles—such as time dilation, quantum tunneling, and material science—this article bridges the fictional flux capacitor with real-world phenomena like Brownian motion, silicon die fabrication, and cathode-ray tube (CRT) technology.
The Flux Capacitor: A Fictional Physics Framework
The flux capacitor’s fictional mechanism inspires speculation within theoretical physics. Although no current scientific theory supports time travel, several concepts align loosely with its description:
1. Time Dilation and Spacetime Manipulation
Einstein’s theory of general relativity provides a foundation for understanding time dilation. Objects traveling near the speed of light experience slower time relative to stationary observers. Theoretically, immense energy densities could distort spacetime enough to create wormholes, shortcuts through spacetime potentially enabling time travel (Morris & Thorne, 1988).
“The curvature of spacetime by mass and energy offers tantalising possibilities, but stabilising such distortions for macroscopic objects remains beyond our capabilities.” (Kip Thorne, Black Holes and Time Warps, 1994)
2. Quantum Tunneling as a Temporal Bridge
Quantum tunneling allows particles to traverse barriers that classical physics would deem insurmountable, a phenomenon critical to semiconductor function (Gurney & Condon, 1928). A speculative device might exploit quantum tunneling to connect disparate points in spacetime.
3. Flux and Capacitor Analogies
The term “flux capacitor” suggests a combination of electric flux—the rate of electric field lines passing through a surface—and capacitors, which store and release electrical energy. In real-world physics, these principles underpin electromagnetic field control and energy storage.
Brownian Motion: Controlled Chaos in Thermal Physics
Brownian motion, observed by Robert Brown in 1827, describes the erratic motion of particles suspended in a fluid, caused by collisions with the fluid’s molecules. Einstein’s explanation (1905) and Perrin’s experimental validation (1908) cemented it as a cornerstone of statistical mechanics.
Applications in Modern Science
1. Nanotechnology: At the nanoscale, Brownian motion becomes a dominant force, influencing molecular interactions and transport mechanisms (Edelstein et al., 2013).
2. Stochastic Processes: The randomness inherent in Brownian motion serves as a model for complex systems, including financial markets and quantum dynamics.
Silicon Die Creation: The Bedrock of Modern Technology
Microchip fabrication exemplifies the precision of material science and quantum mechanics. Silicon dies, the foundation of semiconductors, rely on a multistep process to achieve nanoscale accuracy:
1. Refinement: High-purity silicon is extracted from sand (SiO₂) via carbothermic reduction in electric arc furnaces.
2. Crystal Growth: The Czochralski process produces monocrystalline silicon ingots, essential for uniform electronic properties.
3. Lithography: Ultraviolet light etches intricate circuits onto silicon wafers, pushing the boundaries of Moore’s Law (Moore, 1965).
4. Quantum Effects: At nanoscales, quantum mechanical behaviours dominate, such as electron tunneling and energy quantization, critical for transistor operation (Tsu, 1973).
Cathode-Ray Tubes: A Legacy of Plasma Physics
CRT televisions, a hallmark of 20th-century technology, relied on the manipulation of electrons in vacuum tubes and interactions with phosphorescent materials:
1. Electron Emission: Heated cathodes released electrons through thermionic emission, guided by electric fields to form a focused beam.
2. Phosphors: The electron beam excited phosphorescent materials on the screen, emitting visible light.
3. Gas Discharge: Early CRTs utilised ionised gases to maintain low resistance within tubes, a precursor to modern plasma displays (Spitzer, 1962).
These technologies exemplify the control of energy and matter on both macroscopic and microscopic scales.
Bridging Fiction and Reality: Disciplinary Intersections
To connect the flux capacitor to scientific disciplines, we explore key themes that unite the manipulation of particles and energy across scales:
1. Physics of Controlled Chaos
Brownian motion, CRT plasma physics, and theoretical time machines all involve stochastic processes. Stabilising chaotic systems—whether particle motion in fluids or electrons in a vacuum—remains a central challenge in both physics and engineering.
2. Material Science and Energy Transport
The precise control of energy in semiconductor fabrication parallels the potential requirements of theoretical time-travel devices. Advances in materials like quantum dots or ultrafast transistors could bridge the gap between science fiction and practical energy transport systems.
3. Chemical Physics of Interfaces
Both CRT phosphors and silicon doping rely on the controlled introduction of impurities to alter material properties. These techniques might inform future innovations in materials for time manipulation or advanced semiconductors.
4. Theoretical Physics Meets Technology
Speculative concepts like Bose-Einstein condensates—a state of matter where particles behave as a single quantum entity—might someday underpin technologies akin to the flux capacitor (Anderson et al., 1995).
Conclusion
Exploring fictional devices like the flux capacitor through scientific principles illuminates the intricate interplay of quantum mechanics, statistical physics, and material science. From the chaotic thermal motion of Brownian particles to the precise fabrication of silicon dies and the plasma dynamics of CRTs, a continuum of energy manipulation emerges.
While time travel remains speculative, the pursuit of understanding—driven by curiosity and interdisciplinary connections—may one day transform science fiction into reality. By investigating the boundaries of current knowledge, we pave the way for breakthroughs that blur the line between imagination and innovation.
References
1. Einstein, A. (1905). Investigations on the Theory of the Brownian Movement. Annalen der Physik.
2. Gurney, R. W., & Condon, E. U. (1928). Quantum Mechanics and Radioactive Disintegration. Nature.
3. Moore, G. E. (1965). Cramming More Components onto Integrated Circuits. Electronics Magazine.
4. Morris, M., & Thorne, K. (1988). Wormholes in Spacetime. American Journal of Physics.
5. Spitzer, L. (1962). Physics of Fully Ionized Gases. Interscience Publishers.
6. Tsu, R. (1973). Tunneling in Thin Films. Solid State Electronics.
7. Anderson, M. H., et al. (1995). Observation of Bose-Einstein Condensation in a Dilute Atomic Vapor. Science.
Kommentare